Unlocking Sustainable Futures: The Power of Systems Analysis in Energy Decision-Making
April 23, 2024 | Article by Kelly Walker | Photo composite by Kelly Walker
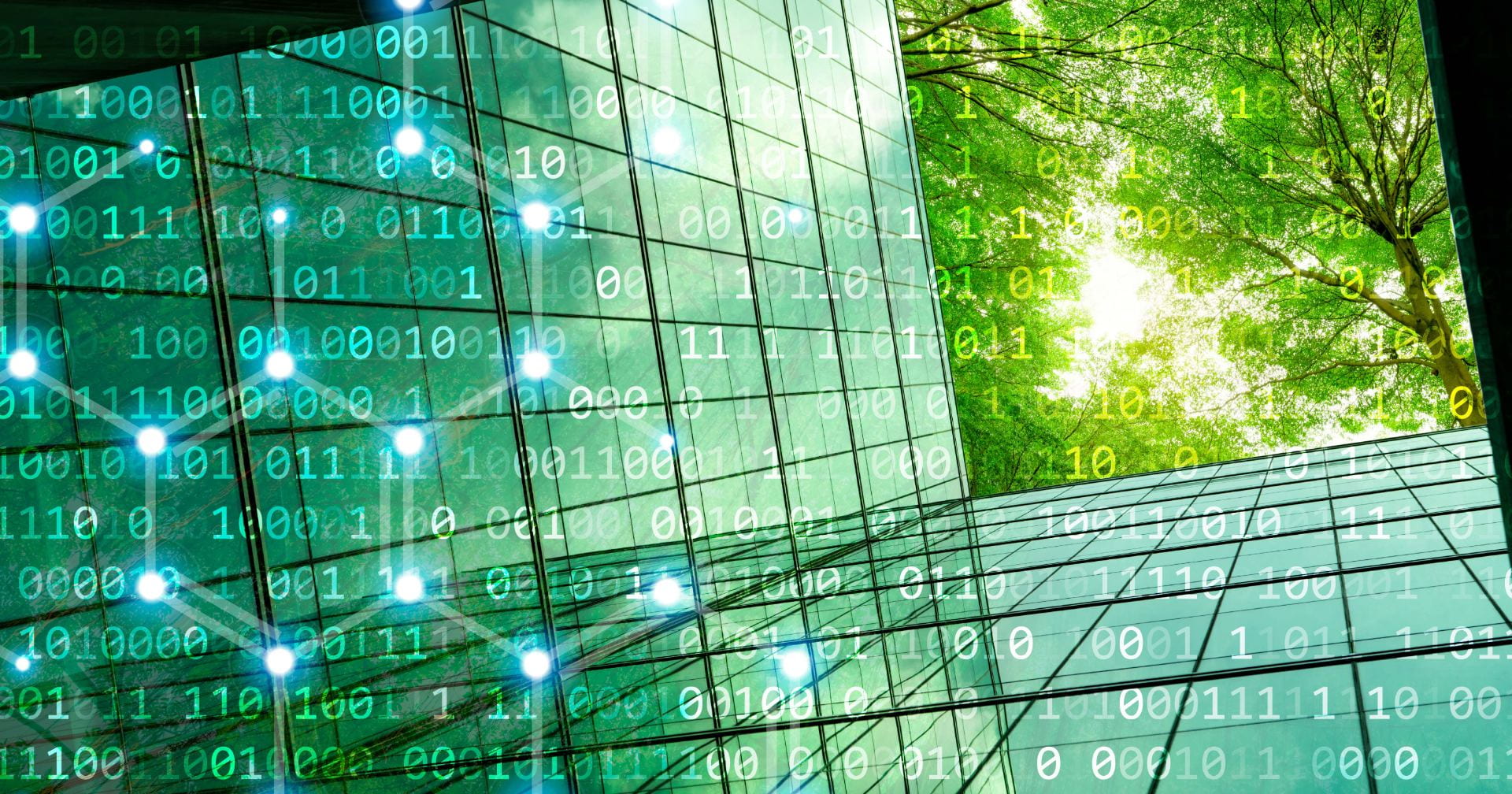
UD researchers are leveraging techno-economic analysis (TEA) and life cycle assessment (LCA) to pinpoint inefficiencies, guide improvements, and optimize processes resulting in tangible economic and environmental benefits — and paving the path for a greener tomorrow.
In a world increasingly focused on sustainability, the intersection of technology and environmental consciousness is crucial. As researchers work to find ways to create more renewable products — using less energy and creating less waste — one team at the University of Delaware is harnessing the power of systems analysis to revolutionize energy and sustainability decision-making.
“We’re looking at how to better utilize available information,” said Marianthi Ierapetritou, UD’s Bob and Jane Gore Centennial Chair of Chemical and Biomolecular Engineering. “It is collaboration between chemical engineering, computer science, and public policy to explore existing literature on renewable products and manufacturing processes, identify knowledge gaps, and develop frameworks to assess the economic, environmental, and market impact of alternative solutions.”
At the heart of this effort lies the utilization of techno-economic analysis (TEA) and life cycle assessment (LCA), two powerful tools that provide invaluable insights into the viability and impact of emerging technologies. TEA allows researchers to accurately analyze the economic aspects of a technology, identify cost contributors, and guide improvements for enhanced efficiency and affordability. LCA provides a comprehensive evaluation of the environmental footprint of a technology or process throughout the entire life cycle of the products, shedding light on factors such as resource consumption, emissions, and ecological impacts. Through detailed research and extensive collaborations, these methods have not only pinpointed bottlenecks but have also guided significant improvements, leading to tangible benefits for both economic and environmental landscapes.
UD researchers have employed TEA and LCA methodologies to reveal inefficiencies that hinder economic viability and environmental sustainability. Consider the case of dimethylbiphenyl (DMBP) production. DMBP, derived from renewable biomass sources, holds promise as a versatile compound in the production of important chemicals. Its production has potential for reduced reliance on fossil fuels and mitigated environmental impact. Through TEA, UD researchers identified long reaction times and high solvent usage as primary contributors of unattractive, high-cost DMBP production. They then optimized reaction conditions – resulting in a remarkable 24% reduction in production costs – and established a sustainable, eco-friendly, and profitable DMBP production technology.
Similarly, in the realm of hydrodeoxygenation reactions, the integration of TEA and LCA within a Bayesian optimization framework proved transformative. By leveraging this approach, the feedback between experimental trials and economic and environmental evaluation is expedited. In a study of the 5-hydroxymethylfurfural hydrodeoxygenation reaction, UD researchers not only increased reaction yields but also identified conditions leading to a 26% reduction in production costs, and a 15% decrease in global warming potentials. Such achievements highlight the synergistic power of analytical methods in driving both economic and environmental efficiency.
TEA and LCA also serve as effective screening tools for promising early-stage technology development and feedstock options. By analyzing cost breakdowns, the potential advantages of alternative methodologies, such as utilizing different reactants or solvents, can be discerned. Through TEA utilization, UD researchers identified glycerin to be a more cost-effective option over methanol for reductive catalytic fractionation (RCF). In this instance, the lignin valorization technology was integrated into a biorefinery that also converts cellulose and hemicellulose components of the yellow poplar feedstock. LCA with the expanded biorefinery system boundary was then used to screen different forest residual feedstocks for environmental benefits, underscoring the pivotal role of these analyses in streamlining processes towards sustainability.
Beyond individual technologies, ensuring optimization across entire supply chains is paramount for implementation of sustainable practices. Here, UD researchers have shown how TEA and LCA play a critical role in guiding decision-making processes. By expanding system boundaries, a comprehensive understanding of factors influencing process viability and scalability can be assessed. Consideration of factors such as policy influences, market volatility, and integration with existing networks uncovers potentials for logistic efficiencies, sustainability improvements, and cost reductions. This holistic approach enables deployment of optimized distribution technologies, minimizes risks, and maximizes sustainability across supply chain networks.
Together, TEA and LCA add to a comprehensive picture of the economic and environmental implications of energy technologies and practices, guiding decision-makers towards more sustainable pathways. The research conducted at the University of Delaware underscores the transformative potential of systems analysis in energy and sustainability decision-making. By harnessing the analytical power of TEA and LCA, researchers are not only optimizing existing processes but also driving innovation towards a more sustainable future. As we navigate the complexities of a rapidly evolving world, such endeavors offer hope and promise for a greener, more prosperous tomorrow.
Read the research:
1. Luo Y, Kuo MJ, Ye M, Lobo R, Ierapetritou M. Comparison of 4,4′-Dimethylbiphenyl from Biomass-Derived Furfural and Oil-Based Resource: Technoeconomic Analysis and Life-Cycle Assessment. Ind. Eng. Chem. Res. 2022;61(25):8963-8972.
2. Wang Z, Luo Y, Srinivasan P, et al. Process integration and systems optimization for the hydrodeoxygenation of 5-hydroxymethylfurfural to dimethylfuran. Comput. Chem. Eng. 2024;184:108644.
3. O’Dea RM, Pranda PA, Luo Y, et al. Ambient-pressure lignin valorization to high-performance polymers by intensified reductive catalytic deconstruction. Sci. Adv. 2022;8(3):eabj7523.
4. Luo Y, O’Dea RM, Gupta Y, et al. A Life Cycle Greenhouse Gas Model of a Yellow Poplar Forest Residue Reductive Catalytic Fractionation Biorefinery. Environ. Eng. Sci. 2022;39(10):821-833.
5. Hernández B, Kots P, Selvam E, Vlachos DG, Ierapetritou MG. Techno-Economic and Life Cycle Analyses of Thermochemical Upcycling Technologies of Low-Density Polyethylene Waste. ACS Sustainable Chem. Eng. 2023;11(18):7170-7181.
6. Selvam E, Kots P, Hernandez B, et al. Plastic waste upgrade to olefins via mild slurry microwave pyrolysis over solid acids. Chemical Engineering Journal. 2023;454.
7. Sahay N, Ierapetritou M. Flexibility assessment and risk management in supply chains. AIChE J. 2015;61(12):4166-4178.
8. Bhosekar, A.; Badejo, O.; Ierapetritou, M. Modular Supply Chain Optimization Considering Demand Uncertainty to Manage Risk. AIChE Journal 2021, n/a (n/a), e17367.